3D Printing
The long road to reliable organ printing
Bioprinted organs could be a revolution in transplantation and regenerative medicine, but a daunting list of scientific challenges stand in the way of the future. What are the main unresolved challenges in the field, and how are academics and the med tech industry working to solve them? Chris Lo finds out.
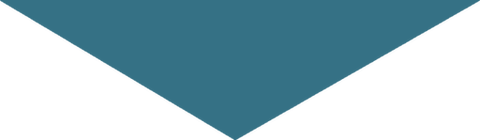
A
s the emerging field of 3D bioprinting continues to develop, it offers the prospect of a true revolution in regenerative medicine. Bioprinting methods are already in use in tissue engineering, organ-on-chip research and the building of organ-shaped constructs of living tissue, among other applications.
Perhaps the ultimate objective in the field is to make functional bioprinted organs a reality for regenerative therapies and transplant purposes. The clinical potential of such a breakthrough is obvious, but so far a host of technical challenges – from cellular density to biomaterial limitations – have left this dream goal just out of reach. The lifecycle limitations of primary cells means that stem cells are the most promising approach for their longevity, self-renewal and pluripotency, so fundamental understanding of stem cell differentiation needs to be improved.
“Despite the successful studies and reported outstanding research efforts, the path to fully build a 3D bioprinted organ has yet to be accomplished and there are several challenges to be solved to further advance this exciting research theme,” wrote Dr Veysi Malkoc of the University of Wisconsin’s Department of Biomedical Engineering in a 2018 editorial published in the Journal of Biomedical Imaging and Bioengineering.
Despite the daunting challenges blocking the path to the first functional 3D-printed organs, this vibrant research area is yielding new innovations every year as academics and the med tech industry work towards overcoming these barriers. Below are some of the most important challenges that remain unresolved in functional organ printing, and recent attempts to address them.
Bioprinters: faster and finer
The incredibly complex process of printing cell-dense 3D structures starts with the bioprinters themselves. These devices – the first of which were adapted from traditional inkjet printers – have modified nozzles allowing for cell-infused bioink to be printed on to a surface in layers. While the current crop of technologies is enabling a range of bioprinting applications and research, they will need a significant bump in resolution, speed and material compatibility to support the journey towards functional organ printing.
Higher resolution will enable better interaction and control in 3D microenvironment.
“Higher resolution will enable better interaction and control in 3D microenvironment,” wrote Malkoc last year. “Currently, printing process is slow so speeding up building the architectures is essential. To reach a commercially acceptable level that is able to mass-produce requires faster printing and scaling up the process.”
New bioprinters being developed are adding extra speed, detail and functionality to help address some of these technical gaps. A recent example comes from the fast-growing biotech start-up Cellink. In September, the firm unveiled its latest bioprinter, dubbed BIO X6, which incorporates six electromagnetic droplet printheads to enable faster printing of complex structures will mixed biomaterials and cell types.
Limited options for biomaterials
The biomaterials that are used to support and give structure to the injected cells that would make up any functional printed organ have a tough task. Synthetic polymers are mechanically strong and adaptable to improve viscosity and printability, but they are non-degradable and can lack the cell adhesion qualities to support adequate cell growth. Natural polymers, meanwhile, are often not as strong as their synthetic counterparts but are much better suited to cell attachment, proliferation and differentiation.
“A blend of these polymers is needed in addition to being compatible with the printing technology,” wrote Malkoc.
Biocompatibility, biodegradability, and mechanical stability are perceived advantages of plant derived materials.
As researchers continue to study materials (and material blends) with just the right properties for bioprinting, avenues are opening up to expand the range of sources for biomaterials.
In April this year, researchers at Swansea published an article in Frontiers in Mechanical Engineering reviewing the promise of plant-based biomaterials in 3D tissue and organ printing. The team highlighted nanocellulose and alginate in particular, noting that plant-based materials combine the strength of plant microarchitecture with the natural benefit of cell growth support.
“Enhanced bioactivity, biocompatibility, biodegradability, and mechanical stability are perceived advantages of plant derived materials, moreover as potential bioinks owing to their affinity for chemical modulation and facile hydrogel formation,” the article observes.
New options for maintaining soft material shape
One of the keys for printing viable organs in three dimensions is maintaining structural integrity, which can be difficult depending on the viscosity of the bioink used. Biodegradable scaffolds made of biomaterials have often been used to maintain the shape of bioprinted tissue and seeding cells, but the drawbacks of scaffolding include provoking immune response, as well as potential toxicity and cell-to-cell interference of degradation byproducts.
These issues have led researchers to look for alternatives, and several projects have presented promising results this year.
In March, researchers at Japan’s Nagasaki University and Saga University published a study on the creation of scaffold-free oesophageal tissue using a multicellular spheroid technique.
Cell-infused biomaterials can then be injected into the gel and built up in layers to create a 3D shape.
A few months later, a team at the University of Illinois at Chicago published their own scaffold-free 3D bioprinting process, using a ‘bath’ of hydrogel beads to allow a bioprinter nozzle to print cells directly without scaffold support. The printing takes place inside the bath of microscopic beads, protecting them before they are hit with UV light to cross-link the beads and create a stable structure for cell growth.
At the University of Birmingham, meanwhile, researchers have taken their own approach to printing soft biomaterials without them sagging or losing their shape. The team’s technique, called Suspended Layer Additive Manufacturing (SLAM), uses a polymer-based hydrogel with particles modified to add self-healing properties. Cell-infused biomaterials can then be injected into the gel and built up in layers to create a 3D shape, with the solution’s protective qualities ensuring that structures can be built precisely and without leaking or sagging.
Mimicking natural vasculature
The body’s natural organs are constantly supplied with oxygen and other nutrients through blood vessels, which also remove waste products. Without this, printed organs will never survive, but the intricacy of natural vasculature is incredibly difficult to mimic with 3D bioprinting – in most cases, the apertures of the nozzles used to print bioink are simply too wide to produce vessels of small enough diameter.
This is another challenge for bioprinter manufacturers, and solutions are starting to emerge. Cellink and Prellis Biologics were recently awarded Merck’s Innovation Award for the Holograph X bioprinter that the partners developed for pharmaceutical research and development.
The intricacy of natural vasculature is incredibly difficult to mimic with 3D bioprinting.
The platform is designed to facilitate in-lab manufacturing of capillary-containing organ structures for transplantation, using ultra-fine resolution and laser-based holographic projection printing.
An academic team at Harvard University’s Wyss Institute for Biologically Inspired Engineering has worked on a new method bioprinting technique called SWIFT (Sacrificial Writing Into Functional Tissue) as another means of creating viable vasculature for bioprinted organs. The technique does so by placing a network of vascular channels into a living matrix of stem cell-derived organ building blocks, creating a structure that mimics the cellular density of human organs while allowing for the supply of nutrients and oxygen through the vascular channels. The channels are created by a thin bioprinting nozzle, which prints the titular ‘sacrificial ink’ into the matrix, moving other cells out of the way without damaging them.