Feature
Industry 4.0 comes to medical devices
Automation in medical device manufacturing is seeing significant digital advances, improving quality, efficiency, cost optimisation, and regulatory compliance, writes Bernard Banga.
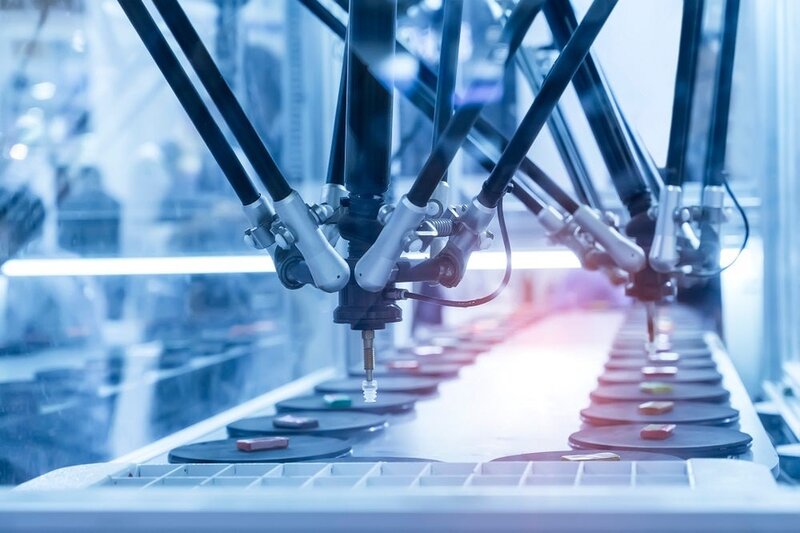
Credit: asharkyu / Shutterstock
Smart factory technology is increasingly being deployed in the manufacture of medical devices. “Thanks to advances in automated manufacturing technology, medical device manufacturers can get closer to achieving zero defects,” claims Raghu Vadlamudi, Chief Research and Technology Director at Donatelle Plastics Inc. This technology minimises variability in raw materials, processes, and assembly, reducing errors which are common with manual operations.
There are four major challenges. Firstly, increasing productivity involves optimising manufacturing processes, identifying bottlenecks in production lines, and automating tasks to achieve higher throughput. Secondly, predictive maintenance must be enhanced through the use of sensors and real-time analytics, so equipment failure and efficient maintenance scheduling can be anticipated. Thirdly, data-driven decision-making is essential for improving resource management and responding to market fluctuations. Lastly, intelligent systems will play a crucial role in optimising energy consumption and minimising waste, thereby reducing environmental impact.
Adaptive robotics using cobots
Collaborative robots (cobots) play a crucial role in integrating smart factories in the manufacture of medical devices. Designed to work alongside human workers, they automate complex tasks and reduce human error. This is particularly notable in healthcare, where precision and efficiency are paramount. Global sales of cobots have been growing rapidly, with 40,000 to 50,000 units sold annually. According to a report from Grand View Research, the global cobot market is projected to reach $12.71 billion by 2030.
The latest generation of cobots features advances in vision systems and precision sensors, enhancing their ability to manipulate complex objects and operate in dynamic environments. Advanced 3D vision systems enable cobots to perceive their surroundings highly accurately, offering micron-level precision, crucial for fine manipulation tasks. High-precision sensors can achieve accuracy of less than 0.1 mm, improving a cobot’s ability to perform delicate tasks through real-time feedback and adjustments.
Modern cobots also incorporate highly sensitive force and torque sensors, allowing safe, precise interaction with humans and delicate objects. Cobots can operate 24/7, significantly boosting production line productivity by up to 30% and reducing defect rates by as much as 20%.
Micro-manufacturing: driving medical device design and functionality
According to Vadlamudi, “rapidly evolving manufacturing technologies, such as micro-manufacturing and 3D printing, are paving the way for groundbreaking innovations in medical device manufacture.” Medical devices and their components are constantly getting smaller, reaching micron dimensions. In 2024, four advanced technologies are at the forefront of micromanufacturing in medical devices. Electron beam lithography stands out for its ability to create structures at nanometre scale with remarkable precision. Plasma etching is being used to sculpt materials at the microscopic level, enabling intricate and detailed patterning. Chemical vapour deposition is being used to deposit thin films onto substrates, producing functional coatings which enhance medical devices. Nanoimprint lithography can transfer patterns at nanometre scale onto surfaces. Lastly, laser micromachining is being used to create precise microstructures in various materials, significantly improving the functionality of medical devices such as stents and implants. These cutting-edge technologies are instrumental in advancing the design and effectiveness of medical devices.
The impact of 3D printing on prototyping, costs, and logistics
Additive manufacturing, commonly known as 3D printing, has been revolutionising the rapid prototyping and cost-effective production of high-resolution, custom components. Key advances in this technology feature four notable innovations. Stereolithography (SLA) stands out, with exceptional resolution and precision, achieving fine detail down to 25 microns. Fused Deposition Modelling (FDM) is popular because of its cost-efficiency and ease of use, achieving resolutions of up to 50 microns. Digital Light Processing (DLP) uses digital light to cure photopolymer resins, offering resolutions which are ideal for detailed prototypes. Large-Scale Photopolymerisation (LSP) leverages light projection to cure extensive areas of photosensitive resin in one step, enabling the production of large, high-resolution parts—particularly useful for fairly large medical devices and anatomical models.
According to a study by Frost & Sullivan, 3D printing significantly reduces manufacturing times for medical devices by 50 to 70%. Prototypes can be produced in days rather than weeks, speeding up the design process. Additionally, 3D printing improves storage and logistics efficiency by enabling on-demand manufacture at the point of use. This approach can cut logistics costs by up to 30%. It also addresses stock obsolescence issues, potentially reducing excess stock levels by 40 to 60%. Finally, the rapid response aspect of 3D printing significantly improves clinical supply chains, further cutting delivery times by 50 to 70% and improving the ability of medical institutions to meet patient needs rapidly.
IoT connectivity combined with big data and AI
In a smart factory, the Internet of Things (IoT) enhances connectivity thanks to real-time data transmission between robots and other devices in the manufacturing chain. “These technologies are advancing automation in manufacturing by enabling real-time data collection and analysis, predictive maintenance, and remote monitoring,” claims Vadlamudi. One key aspect of Industry 4.0 in medical device manufacturing, the integration of advanced IoT sensors, reduces unscheduled downtime by up to 30% through optimised predictive maintenance. IoT also helps reduce energy consumption by 10 to 20%.
Artificial Intelligence (AI) and Machine Learning (ML) are transforming medical device manufacturing by analysing extensive datasets to reveal patterns, predict trends, and make data-driven decisions. AI is increasingly being used for modelling, numerical simulation, and optimising production yields. It adeptly manages time-series data, including continuous measurement and historical performance records for equipment, alongside data from IoT sensors, product quality metrics (such as precision measurements, defect rates, and compliance test results), and performance indicators such as yield rates, downtime, and production cycles. AI-driven automation enhances predictive maintenance by analysing sensor data from manufacturing equipment, improves quality control with precision surpassing human inspectors, identifies process inefficiencies, and refines design and development to produce designs better suited for automated manufacturing. Numerous studies have highlighted and quantified the significant impact of automation and AI on manufacturing processes in 2024. For instance, the latest report from Deloitte on manufacturing productivity indicates that automation and AI technology can boost productivity by between 25% and 40%, by optimising manufacturing processes and reducing downtime. We are well on the say to seeing next-generation smart factories in medical device manufacture.
Four key challenges in integrating automation technology into medical device manufacture
- High initial investment: Implementing advanced automation systems requires significant capital investment. This includes the cost of new equipment, software, and training.
- Specialist expertise: Designing, implementing and maintaining complex automated systems require specialist skills. There is often a shortage of skilled professionals who can manage this advanced technology.
- Flexibility and adaptability: Automated systems need to be flexible enough to adapt to changes in product design and regulatory requirements.
- Integration with legacy systems: Many medical device manufacturers have existing legacy systems which need to be upgraded. This can be complex and may require significant modifications to existing processes.
Five potential risks of integrating AI into medical device automation
- Data privacy and security: AI systems require large amounts of data, which can include sensitive patient information. Ensuring the privacy and security of this data is a significant challenge, as breaches could lead to severe consequences.
- Bias and fairness: AI algorithms can inherit bias present in the training data, leading to unfair or inaccurate outcomes. This is particularly critical in medical applications.
- Overfitting and false associations: AI models can sometimes overfit the training data, identifying patterns that do not generalise well to new data. This can lead to false associations and potentially harmful decisions in medical device manufacturing.
- Regulatory compliance: Ensuring that AI systems comply with stringent regulatory standards is complex. The lack of clear guidelines for AI in medical device manufacturing can represent risks during the approval and validation processes.
- Technical failure: AI systems are susceptible to technical failure, which can disrupt manufacturing processes and lead to defective products. Ensuring robust and reliable AI systems is crucial in mitigating this risk.
“We do this all virtually on the computer, so we can make the osteotomy in multiple different places to decide where the most appropriate place to do the correction is.”
From here, relevant standard orthopaedic plates are selected for use in the surgery.
Following these preliminaries, surgical guides, jigs, and plastic models of the patient’s anatomy, in this first case the radius, are 3D printed and then sterilised for use in surgery.
“We make sure that the guide fits the bone in the patient exactly the way we planned for it to fit on the plastic bone. Once we have made sure that’s the case, we secure the guide to the bone with wires, and then we do whatever the plan has been,” says Lattanza.
In osteotomy, such plans generally involve drilling holes and then making the necessary bone cuts.
The great thing about this approach, Lattanza states, is that all that needs to be done to ensure the correction has been completed as planned during the surgery is to line up those holes.
She explains: “If the bone is rotated off 90° and when we drill those holes, they’re off 90° on the bone, we make the cut then we rotate and line up those holes to put the plate on because the plate holes are straight, and that’s how we know that we’ve got the correction.”
Beyond making relatively common osteotomies more accurate, a 3D provision also allows for more complex cases to be worked upon. Lattanza relays a recent case in which a child had broken the radius and ulna bones in their forearm.
“During the time that she was growing, this deformity got ‘very 3D’, meaning it was off in the sagittal, coronal, and axial plane,” says Lattanza.
“You can’t see the axial plane on an X-ray, and if you can’t see it, you can’t correct it.”
In this case, the procedure required two cuts in the radius to restore it to normal anatomy, and one in the ulna.
“In my career prior to having the 3D technology, that’s something that is difficult or impossible to plan and to execute in the operating room, because you wouldn’t even be able to see that you needed two cuts to make it normal again,” explains Lattanza.
Lattanza is keen to add that the influence of 3D printing on preoperative planning and during surgery should not be a cause for complacency, particularly given that there remain limitations to 3D visualisations of CT scans, chiefly in that the current technology cannot show soft tissue.
“Some people think that this is kind of a phone it in now, but that’s not how it works,” she says.
“This is a collaboration between an engineer and a surgeon, and it has to be that way to get a good result.”
Once we see where those changes are, we can plan where we’re going to cut the bone.
Dr Lattanza
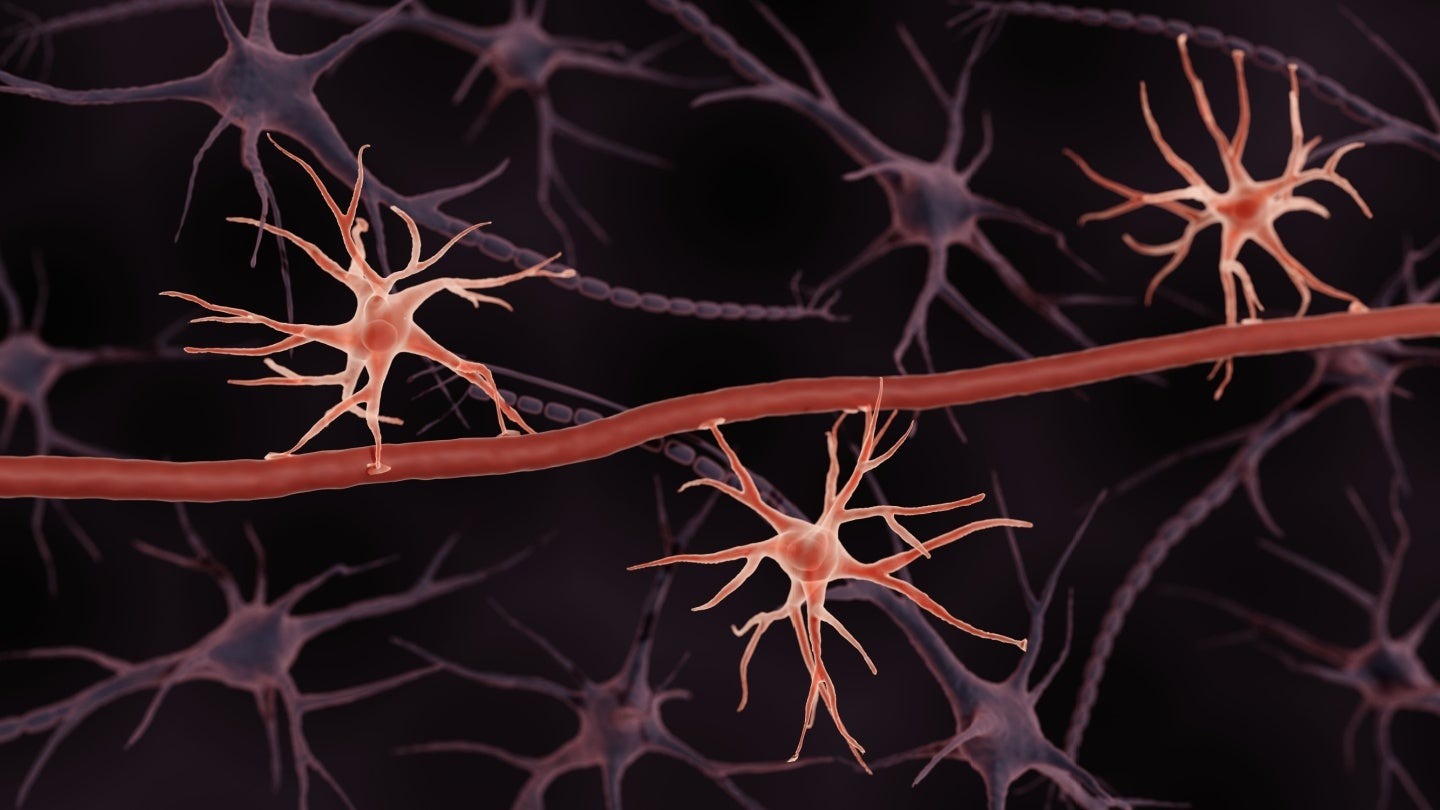
Astrocytes are a type of neural cell that builds the BBB, and Excellio plans to derive exosomes from them to make them even better at targeting the brain. Credit: ART-ur / Shutterstock
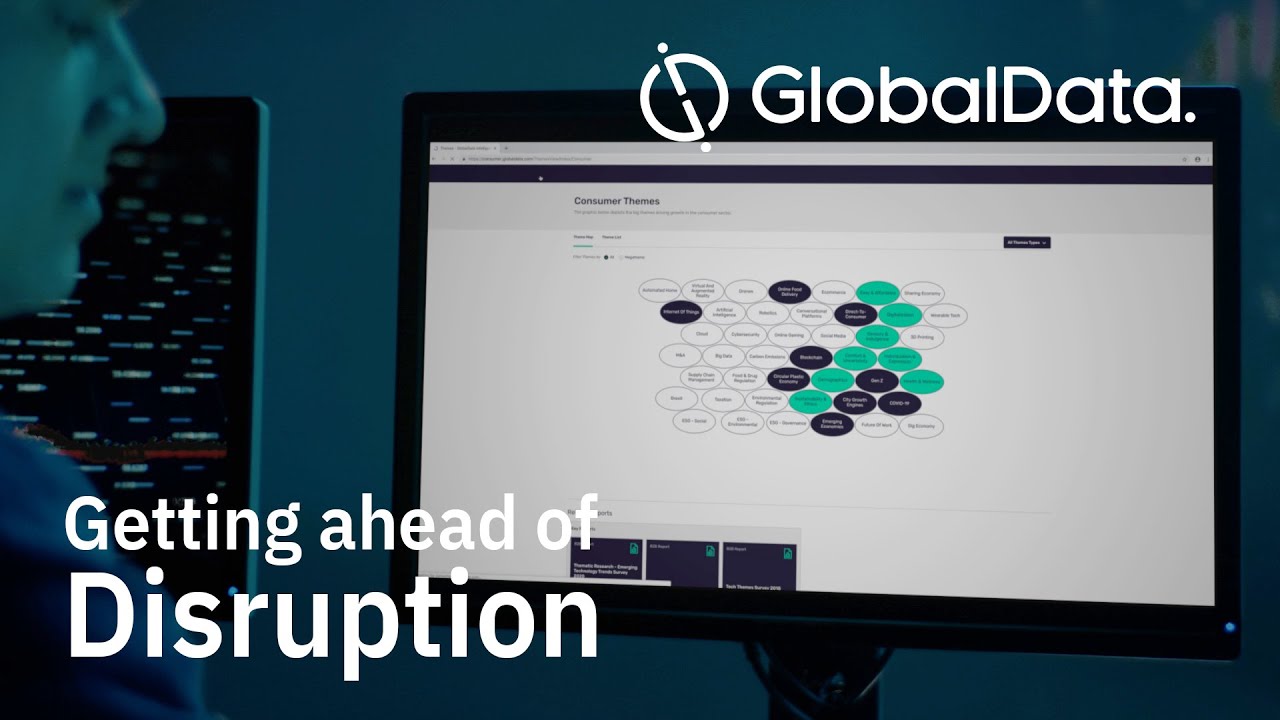
Caption. Credit:
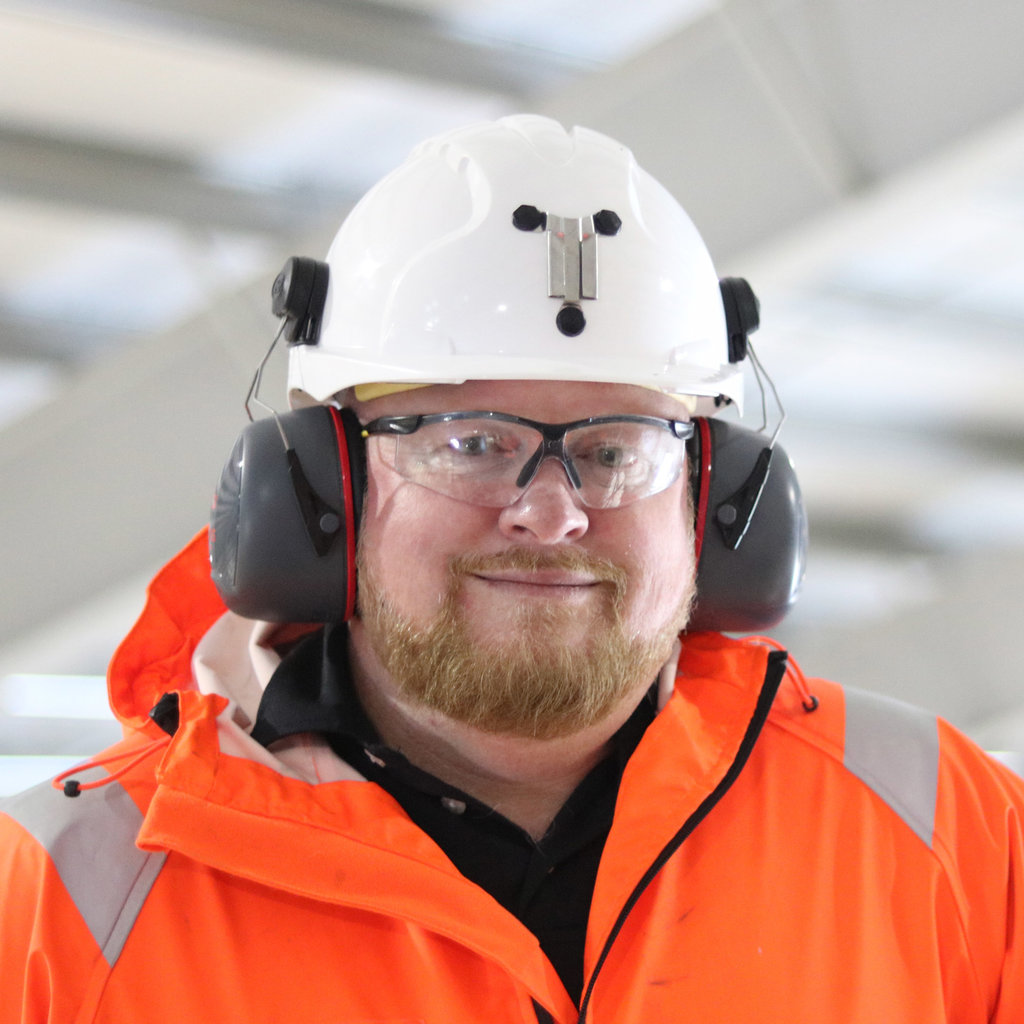
Phillip Day. Credit: Scotgold Resources
Total annual production
Australia could be one of the main beneficiaries of this dramatic increase in demand, where private companies and local governments alike are eager to expand the country’s nascent rare earths production. In 2021, Australia produced the fourth-most rare earths in the world. It’s total annual production of 19,958 tonnes remains significantly less than the mammoth 152,407 tonnes produced by China, but a dramatic improvement over the 1,995 tonnes produced domestically in 2011.
The dominance of China in the rare earths space has also encouraged other countries, notably the US, to look further afield for rare earth deposits to diversify their supply of the increasingly vital minerals. With the US eager to ringfence rare earth production within its allies as part of the Inflation Reduction Act, including potentially allowing the Department of Defense to invest in Australian rare earths, there could be an unexpected windfall for Australian rare earths producers.