Sensors
Sensors and sensitivity
Sensors are used for a wide range of applications in healthcare, from biomarker-measuring wearables to disease diagnostics. As the devices used to capture this data become more complex, sensors have become a prime area for innovation. Chloe Kent rounds up some of the ways manufacturers are developing novel sensing technologies.
T
he challenge of providing quality healthcare while complying with Covid-19 restrictions has lit a fire under the remote monitoring and telehealth market. In turn, this has led to an increase in research and development into technologies that can gather readings on a person’s health without a clinician ever needing to be in the same room.
Alongside developments in conventional sensing technology, several research groups around the world have been working to develop sensors with a twist. Whether they’re fashioned from unconventional materials or freeze-dried into fabrics, the world of sensor technology has never been stranger.
Medical Technology takes a look at some of the most innovative novel sensing technologies currently under development.
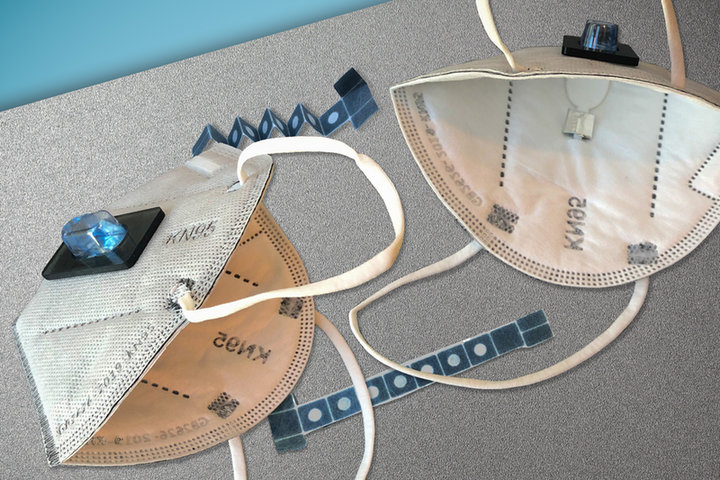
Credit: Wyss Institute at Harvard University
Sensors in a facemask can diagnose Covid-19
Engineers at the Massachusetts Institute of Technology (MIT) and Harvard University have designed a novel face mask with sensors that are embedded into the fabric, which can diagnose the wearer with Covid-19 in just 90 minutes.
Designs for the disposable sensors are based on freeze-dried cellular machinery that the research team previously used when developing paper diagnostic technologies for viruses, such as Ebola and Zika. They have now demonstrated that the sensors can be incorporated into fabrics, offering a potential new way to monitor healthcare worker’s exposure to pathogens while on shift.
The sensors build upon a technology developed in 2014 by MIT professor of medical engineering James Collins. He showed that proteins and nucleic acids create synthetic gene networks that react to specific target molecules that could be embedded into paper. These components were further refined in 2017, into a CRISPR-based technology known as SHERLOCK, which allows for the highly sensitive detection of nucleic acids.
These components can be freeze-dried and remain stable for months on end until they are activated by liquid. After that, they can interact with their target DNA or RNA sequence – for example, Covid-19 antigens – and change colour if the substance is detected. When embedded into a synthetic fabric, the sensors are surrounded by a ring of silicone to keep the samples from evaporating or diffusing away from the sensor.
To produce the diagnostic face mask, researchers embedded the freeze-dried SHERLOCK sensors into the inside of a paper mask, so they can detect viral particles in the breath of an infected wearer.
The sensors are activated by the wearer using a built-in reservoir of water that hydrates the freeze-dried components once they are ready to perform the test. To ensure their privacy, the results are only displayed on the inside of the mask.
The researchers claim that this test is as sensitive as the gold-standard Covid-19 diagnostic, a polymerase chain reaction test. As well as the insides of masks, they have also embedded the sensors on the outside of clothing to detect Covid-19 exposure in the environment.
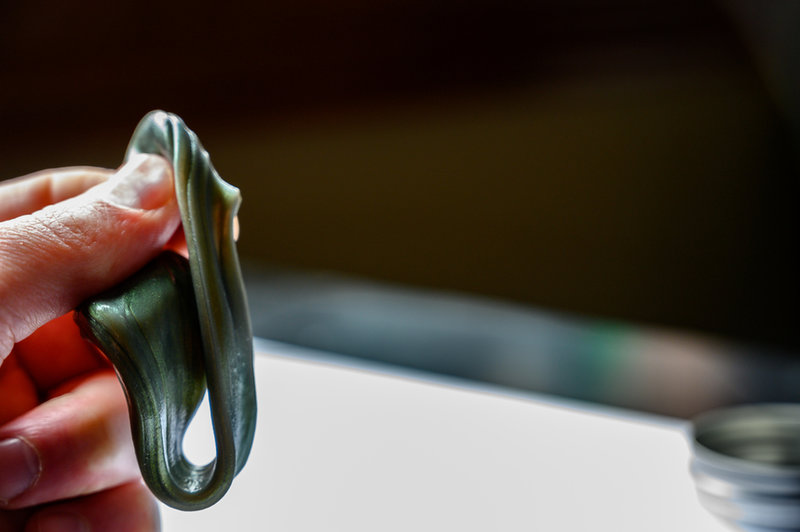
G-putty: silly putty as a strain sensor
In 2016, Graphene Flagship researchers from Trinity College Dublin collaborated with the National Graphene Institute and the University of Manchester to create an extremely sensitive sensor by engineering the children’s novelty toy Silly Putty to conduct electricity.
By fusing the polysilicone in the putty with graphene, the electrical resistance of the product became extremely sensitive to the slightest deformation or impact. When mounted onto the head or neck of human subjects, the substance could be used to measure breathing, pulse and blood pressure. It also showed unprecedented sensitivity as a sensor for strain and pressure and as an impact sensor.
Christened the G-putty, researchers at AMBER, the SFI Centre for Advanced Materials and BioEngineering Research and Trinity’s School of Physicians have developed a next-generation sensing technology from the material.
The research team developed a method to formulate G-putty based inks, creating and testing inks of various viscosities depending on printing technology used and intended final application. They found that the G-putty could be printed as a thin film onto elastic substrates, such as plasters, and attached to the skin.
The printed sensors are 50 times more sensitive than the industry standard. Perhaps more importantly, they outperform other comparable nano-enabled sensors in terms of flexibility, which could be a game-changer for the research team.
G-putty has been able to measure several different bodily functions but could excel as a strain sensor. Strain sensors work by detecting mechanical change and converting it into a proportional electrical signal.
They can be highly valuable diagnostic tools, for example by helping detect changes in the ability to swallow after a stroke. However, the strain sensors currently on the market are predominantly made from metal foil that poses limitations in terms of wearability, versatility and sensitivity.
Trinity College Dublin professor of chemical physics Jonathan Coleman, who has led the G-putty research, was recently awarded a European Research Council Proof of Concept grant to build upon these findings and develop a prototype for a commercial product.
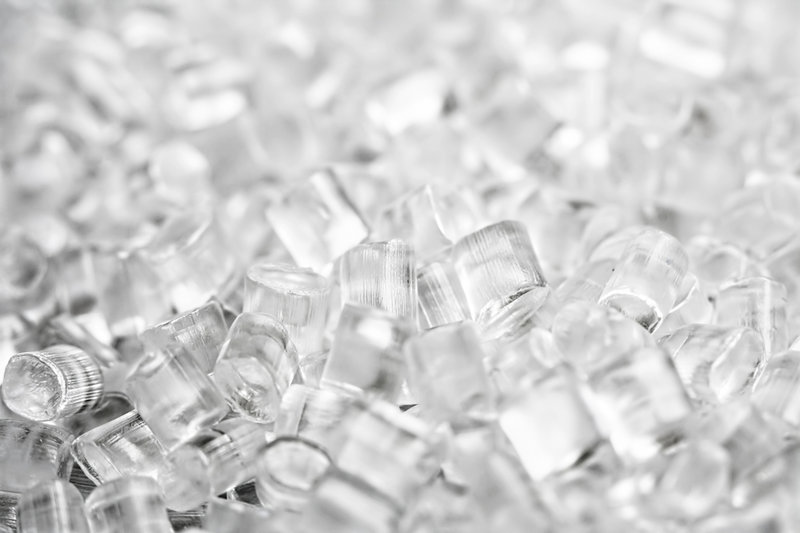
Railway monitoring fibreoptics repurposed for the human body
Advanced railway safety monitoring technology could one day be used to monitor our health. Researchers at Hong Kong Polytechnic University (PolyU) have repurposed this technology to create novel fibreoptic microsensors that are biocompatible, supple and sensitive to even small pressure changes inside the human body.
To deploy this optic fibre technology inside the human body, the team had to refashion it from its industrial form. Traditional optical fibres are made of glass or plastic, materials that can have significant drawbacks when implanted inside the human body. Glass is too stiff and brittle, while plastic fibres can be prone to absorbing water.
The PolyU team have solved these problems by basing their fibreoptic sensors in an advanced plastic material called ZEONEX. This material is also made more sensitive by adding a side hole running in parallel with the light transmission path inside the optical fibre.
ZEONEX isn’t sensitive to humidity or chemical changes and is supple and shatter resistant. It can also be made as small as a few micrometres in size, making it ideal for integration with many medical implants.
The material also has a sensitivity to pressure 20 times that of traditional optic sensors, enough to even measure pressure changes in the lungs while breathing.
The team are now exploring the use of the sensors for precise navigation and shape detection during cardiac catheterisation and seeing if they can expand their measurement abilities to other physiological changes like acidity and temperature.
They are also working with other institutions to integrate its sensor with medical products.
The team is working with the University of Melbourne to integrate the sensors inside a smart cochlear implant, which could provide critical information about location and force to surgeons in real-time during the implantation procedure. They are also working with Monash University to integrate the sensors into orthopaedics implants for monitoring bone and fracture recovery.
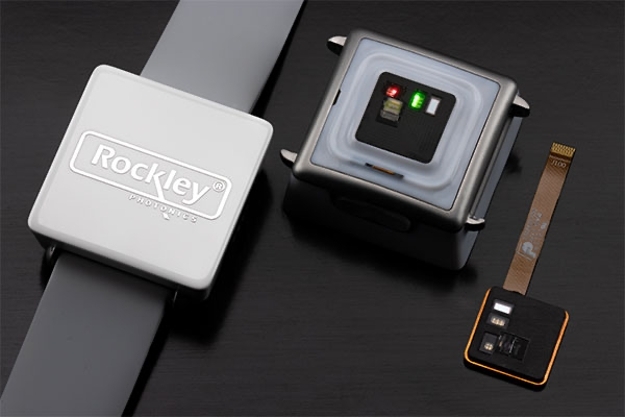
Image: The photonic clinic-on-the-wrist device developed by Rockley Photonics. Credit Rockley Photonics
Rockley Photonics unveils spectrophotometer on a chip
Rockley Photonics, a company long-rumoured to be reinventing the Apple watch, has unveiled a photonic clinic-on-the-wrist device that integrates hardware, application firmware and cloud analytics into a single wearable.
The wristband is designed to monitor multiple biomarkers, including core body temperature, blood pressure, body hydration, alcohol, lactate and glucose trends, among others. It will be trialled in a series of in-house human studies in the coming months.
Rockley designs silicon photonic sensors called spectrophotometers, which can monitor a person’s blood using infrared light. The sensors, which the company claims are significantly more accurate than the LED ones commonly used in smart wearables, are designed to continuously monitor biomarkers that can usually only be tracked with invasive medical equipment.
Unlike more common spectroscopy solutions, which use broad-spectrum light sources, Rockley’s sensor module generates numerous discrete laser outputs from a single silicon chip covering a broad optical band.
The sensor non-invasively probes beneath the skin to analyse blood, interstitial fluids and various layers of the dermis for biomarker changes. It delivers several milliwatts of optical output power per wavelength channel, which is key to achieving the high signal-to-noise ratio required for signal analysis from such a small wearable.
The company is initially targeting the consumer electronics market but plans to pursue the application of its technologies with medical device companies in the future.