Feature
Advancing diabetes: The next-gen implantable bioartificial pancreas
MIT researchers are developing wireless, battery-free oxygen-generating device for immune isolation of transplanted insulin-producing cells in diabetes treatment. By Bernard Banga.
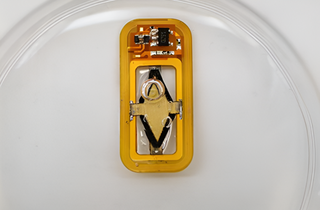
Bioartificial pancreas, diabetes. Credit: MIT
In patients with type 1 diabetes, the pancreas stops producing insulin, the hormone essential for regulating blood sugar levels. According to estimates from the International Diabetes Federation (IDF), type 1 diabetes affects 8.42 million people worldwide, with 510,000 new cases diagnosed every year. Most of these patients have to meticulously monitor their blood glucose levels and administer insulin injections at least once a day. However, this method fails to replicate the body’s natural glucose control mechanism.
An alternative approach involves transplanting pancreatic islet cells into the liver. These cells can detect blood sugar levels and release insulin as needed. But such transplants have limitations.
“Patients require immunosuppressive therapy to prevent rejection, and a portion of the transplanted cells may perish within days due to insufficient oxygen supply”, explains Daniel Anderson, Professor of Chemical Engineering at Massachusetts Institute of Technology (MIT).
Wirelessly powered technology using proton-exchange membrane
To address this dual challenge, a team from MIT, in collaboration with researchers from Boston Children’s Hospital, has developed a novel implantable device. The O2-Macrodevice not only carries hundreds of thousands of islet cells but is also able to generate its own oxygen supply by splitting water vapor present in the body.
The research behind this innovation was supported by funding from the Juvenile Diabetes Research Foundation (JDRF), the Leona M. and Harry B. Helmsley Charitable Trust and the National Institute of Biomedical Imaging and Bioengineering (NIBIB) at the National Institutes of Health.
“The system relies on electrochemical water splitting based on a water-vapor reactant feed, sustained by wireless power harvesting based on a flexible resonant inductive coupling circuit”, explained MIT Research Scientist Siddarth Krishnan. “This is done using a proton-exchange membrane – a technology originally developed to generate hydrogen in fuel cells – located within the device”.
Water vapor, which is present in abundant quantities in the body, is split by the membrane into hydrogen, which diffuses safely away, and oxygen. The oxygen is then stored in a chamber and delivered to the islet cells via a thin, oxygen-permeable membrane. One of the major benefits of this approach is that it does not need any wires or batteries.
Splitting the water vapor requires a small voltage (about 2 volts) that is generated by resonant inductive coupling, a phenomenon commonly used to charge wireless devices. A tuned magnetic coil located outside the body wirelessly powers a small flexible antenna within the device. This method requires an external coil, which the researchers suggest might be worn by patients as a skin patch. “The device does not require pumping, refilling, or ports for recharging and does not generate potentially toxic side products,” stated Krishnan.
Implantable oxygen device regulates blood glucose in mice
“Through systematic in vitro studies with primary cell lines and cell lines engineered to secrete protein, we demonstrate device performance in preventing hypoxia in ambient oxygen concentrations as low as 0.5%,” said Krishnan. The MIT researchers demonstrated the system’s potential to support subcutaneous survival of encapsulated islet cells in awake, freely moving animals with a fully functional immune system in tests conducted in diabetic mice. One group was implanted with the oxygen-generating device, while a control group received a device containing islet cells without supplemental oxygen.
The results of this study, published in Proceedings of the National Academy of Sciences (PNAS), showed that mice implanted with the oxygen-generating device maintained normal blood glucose levels for up to a month. The system thus enables islet cells to survive and function at high densities, around 1000 islets per cm2, in vivo without immune suppression, producing normal blood glucose levels in diabetic animal models.
Looking towards in-human trials and other therapeutic areas
This study marks a significant initial step towards conducting preclinical tests on larger mammals, including humans. The next phase will involve enhancing cell density within the implant while maintaining its compact size. The MIT research team aims to develop a larger version of this device, similar in size to a stick of chewing gum, for testing in individuals with type 1 diabetes.
However, since powering the electrolyser will require hundreds of milliwatts, it will need to integrate an externally rechargeable, potentially rather bulky implantable battery. This would introduce a new dependency for the patient, not on insulin but on electrical energy, as noted by Abdelkader Zebda, a research fellow at the French National Centre for Scientific Research (CNRS) ‘Techniques of Medical Engineering and Complexity – Computing, Mathematics, and Applications’ (TIMC-Imag) laboratory at Grenoble University Hospitals.
According to Zebda, a multidisciplinary expert on the use of artificial pancreas technology in diabetes, it will also be important to prevent fibrosis (tissue changes caused by surgical intervention) from occurring around the implantable device. This means that it will be necessary to develop materials that prevent the accumulation of biological matter and are minimally biodegradable over time, added Zebda.
Meanwhile, the MIT researchers believe that their O2-Macrodevice could potentially be used in other diseases requiring repeated therapeutic protein delivery over extended periods. For example, their research has also demonstrated the ability of the device to sustain cells producing erythropoietin, a protein stimulating red blood cell production.
“We’re optimistic that it will be possible to make living medical devices that can reside in the body and produce drugs as needed”, remarked Anderson, concluding that there are a variety of diseases where patients need to take proteins exogenously, sometimes very frequently. “If we can replace the need for infusions every other week with a single implant that can act for a long time, I think that could really help a lot of patients.”
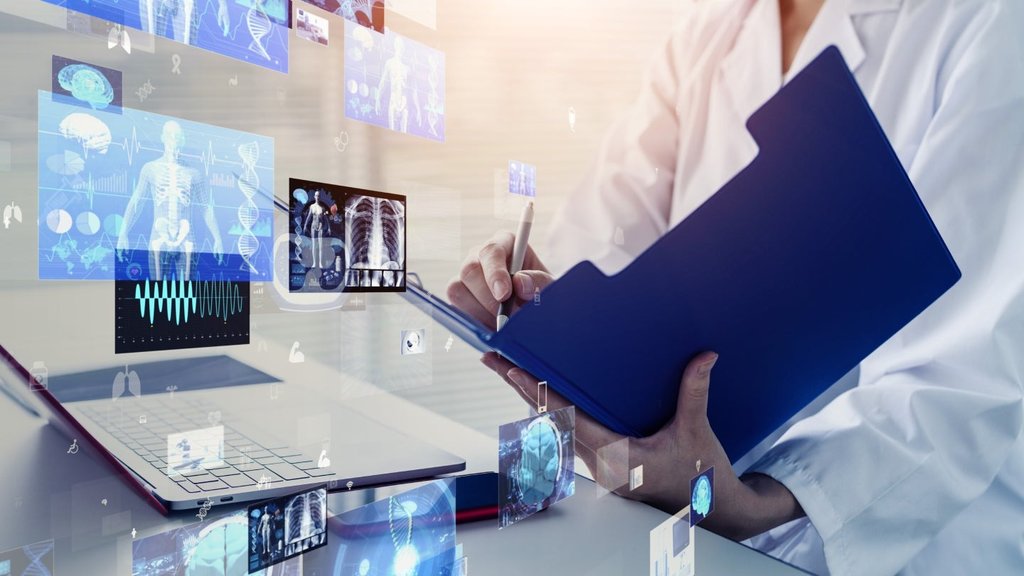
Caption: The US Pentagon is seeking to reduce carbon emissions through a range of programmes, but will it go far enough? Credit: US DoD
Total annual production
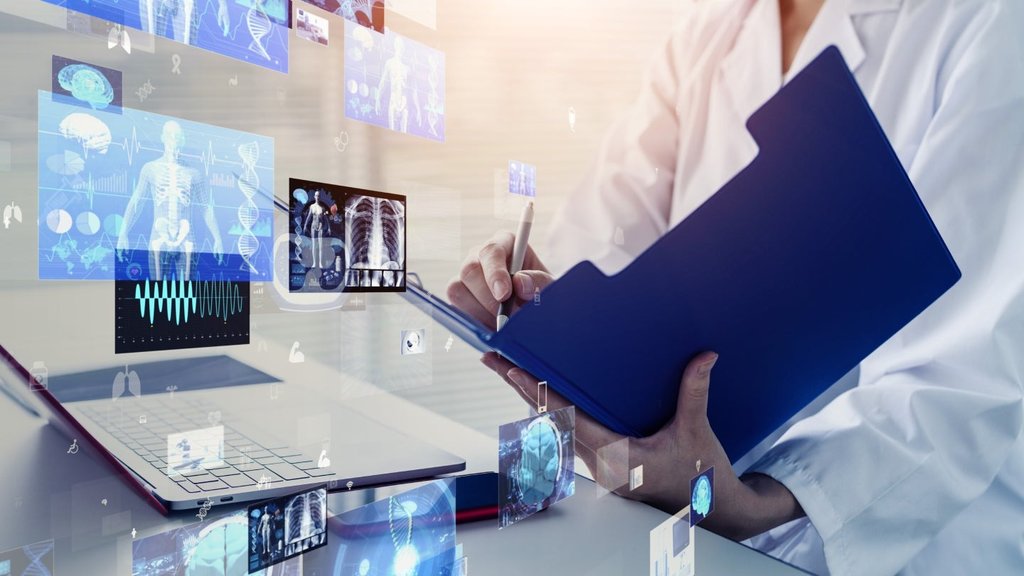
$345m: Lynas Rare Earth's planned investment into Mount Weld.
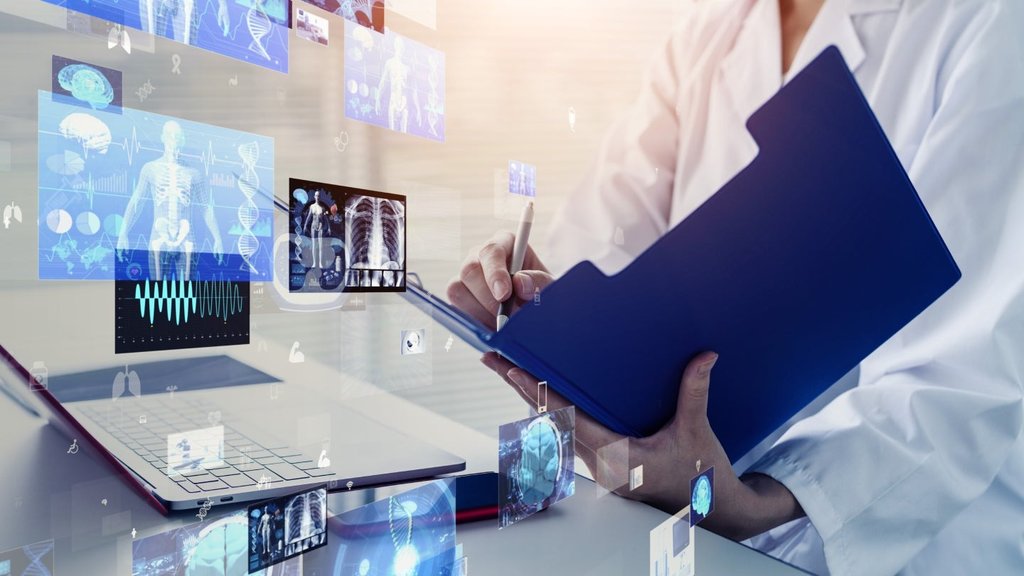
Caption. Credit:
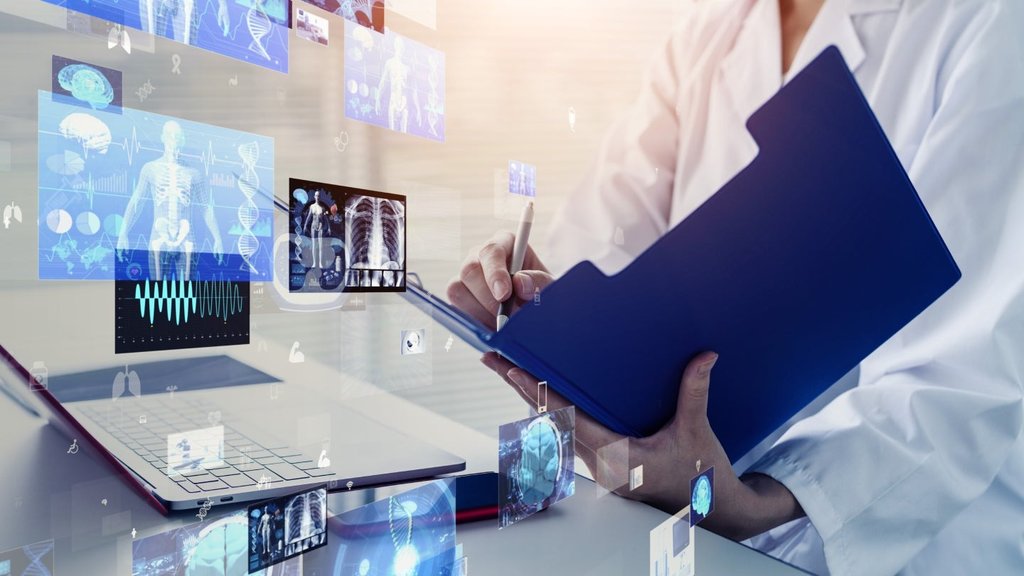
Phillip Day. Credit: Scotgold Resources